Secrets for Solar Orbiter to solve
Every morning the Sun rises and bathes the Earth in life-giving heat and light. It is a true constant in our lives, and has been this way for aeons. Yet there are deep mysteries that we have yet to understand about the Sun. As important as these mysteries are for advancing our scientific knowledge of the Sun and the wider universe, there are also practical reasons for wanting to understand the Sun’s behaviour more deeply.
As we become more reliant on technology, and especially satellites, to help us conduct our daily lives, we are going to have to understand the Sun and the way it can affect that technology in order to build reliable systems. If we are truly going to explore the Solar System with astronauts then again, we are going to have to recognise the Sun’s behaviour to keep those crews safe from dangerous radiation. This all means that there is lots of work for Solar Orbiter to do.
How does the Sun’s magnetic field affect Earth?
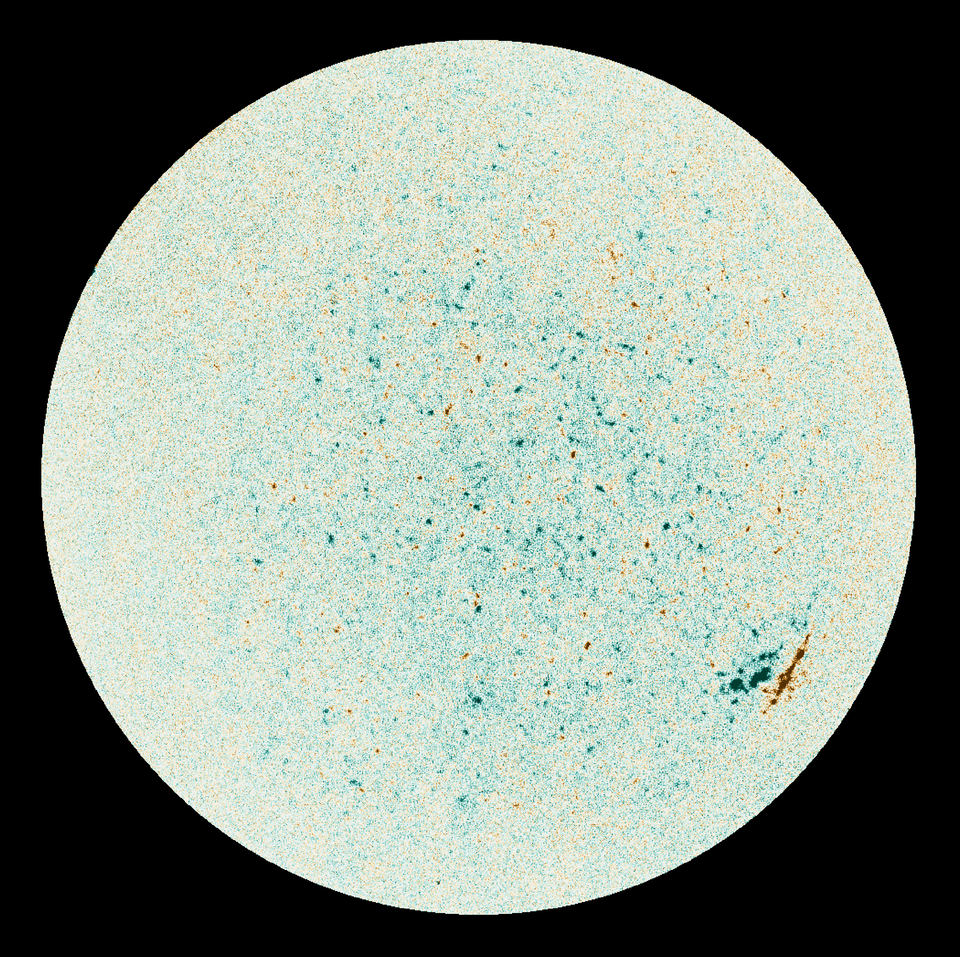
Despite the Sun’s nearly constant output of infrared and visible light, our nearest star is variable at other wavelengths and this is where Solar Orbiter is concentrating its investigations. The variability tends to be most pronounced at ultraviolet and X-ray wavelengths, which are shorter than the visible light we can see with our own eyes. In the ultraviolet and X-ray regions of the electromagnetic spectrum, the Sun can alter the quantity of radiation it emits by factors of ten, hundreds or in extreme cases thousands. These mindboggling variations are caused exclusively by solar magnetic activity.
It has been recognised for more than a century and a half that the Sun is capable of transmitting electromagnetic energy across space that can impact technology on Earth. In 1850, it was noticed that an unexplained daily drift in the compass needles on Earth correlated to the average number of sunspots on the Sun. Puzzling over the implication that the Sun must therefore be magnetic, Sir John Herschel wrote to Michael Faraday, who was experimenting with electricity and magnetism and stated: “If all this be not premature we stand on the verge of a vast cosmical discovery such as nothing hitherto imagined can compare with.”
The discovery was indeed correct, and the study of the Sun’s electromagnetic links to Earth has only grown in importance during the last 170 years. ESA’s Solar Orbiter is designed to elevate this investigation to maturity by looking in unprecedented detail at how the Sun generates and propagates its magnetic field out into space, and the sources of variability in that generation.
What drives the Solar dynamo?
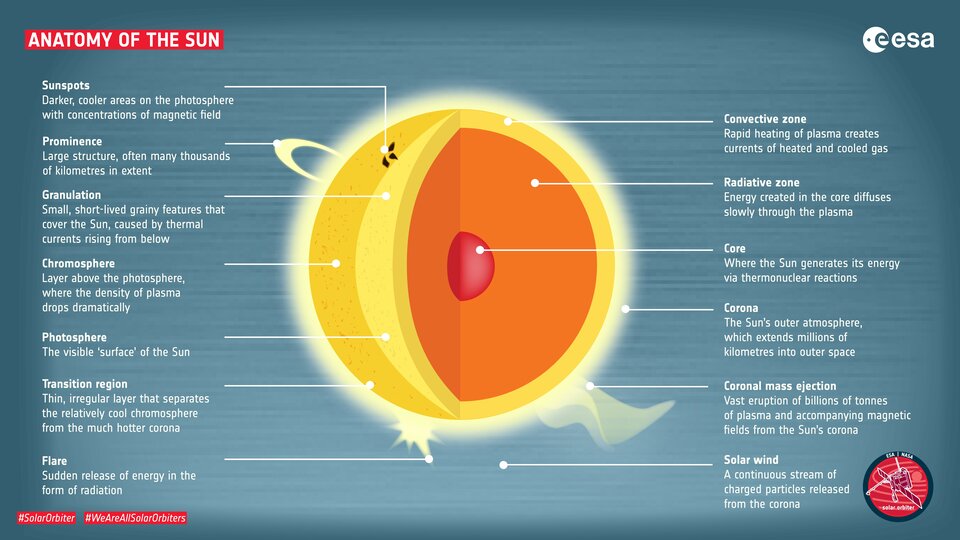
The Sun’s magnetic field is responsible for all the solar activity we see; it drives the 11-year ebb and flow in the number of sunspots, and dominates the behaviour of the solar atmosphere. Furthermore, this magnetic field surrounds the entire solar system, creating a giant bubble known as the heliosphere that is filled with electrified gas known as plasma. Disturbances in this plasma can affect the planets, creating aurorae and interfering with the behaviour of technology. Yet the details of the magnetic field’s initial generation inside the Sun are not yet understood.
“We know that the magnetic field is responsible for all the activity that the Sun produces but we don’t know how the magnetic field is produced,” says Sami Solanki, the director of the Max Planck Institute for Solar System Research in Göttingen, Germany, and Principal Investigator of the Polarimetric and Helioseismic Imager (PHI), one of the instruments on Solar Orbiter. “We think it’s a dynamo inside the Sun that is doing it, similar to the dynamo that produces Earth’s magnetic field, but we really don’t know how the Sun’s dynamo functions.” Solar Orbiter will address this gap in our knowledge by investigating the initial generation of the magnetic field, deep inside the Sun. But how can we study all of this when we can’t see below the Sun’s surface and the magnetic fields that do reach out are invisible to the naked eye? This is where PHI comes into its own.
The instrument looks at a specific wavelength of light, known as a spectral line, that is emitted by atoms of iron contained in the Sun. In the presence of a magnetic field, the iron atom is deformed and the spectral line is split into several components by a phenomenon called the Zeeman effect. The scale of the splitting allows the team to measure the strength of the magnetic field. A second property of the line, called polarization, is also measured by PHI as this contains information about the direction of the magnetic field.
“We have to analyse the light that we get from the Sun in all aspects. It is a very complex optical system that we have put into this instrument,” says Achim Gandorfer, Max Planck Institute for Solar System Research (MPS), and a PHI team member.
PHI can also ‘look’ below the Sun’s surface. The solar surface is in constant motion up and down because convection and turbulence below the surface create the equivalent of seismic waves that propagate through the Sun’s interior. PHI will measure these motions to unprecedented levels of precision, allowing scientists to run computer models of the solar interior to see what conditions allow the waves that they see to take place.
This is important for understanding how the magnetic field is generated because theorists believe that the Sun’s magnetic field originates in a region of the Sun’s interior called the tachocline. This is the layer about 30 percent of the way inside the Sun, where the properties of the Sun’s rotation change dramatically, leading to great shearing forces in the plasma there. The movement this causes in the plasma generates the magnetic field that then rises to the Sun’s surface.
What’s going on at the poles?
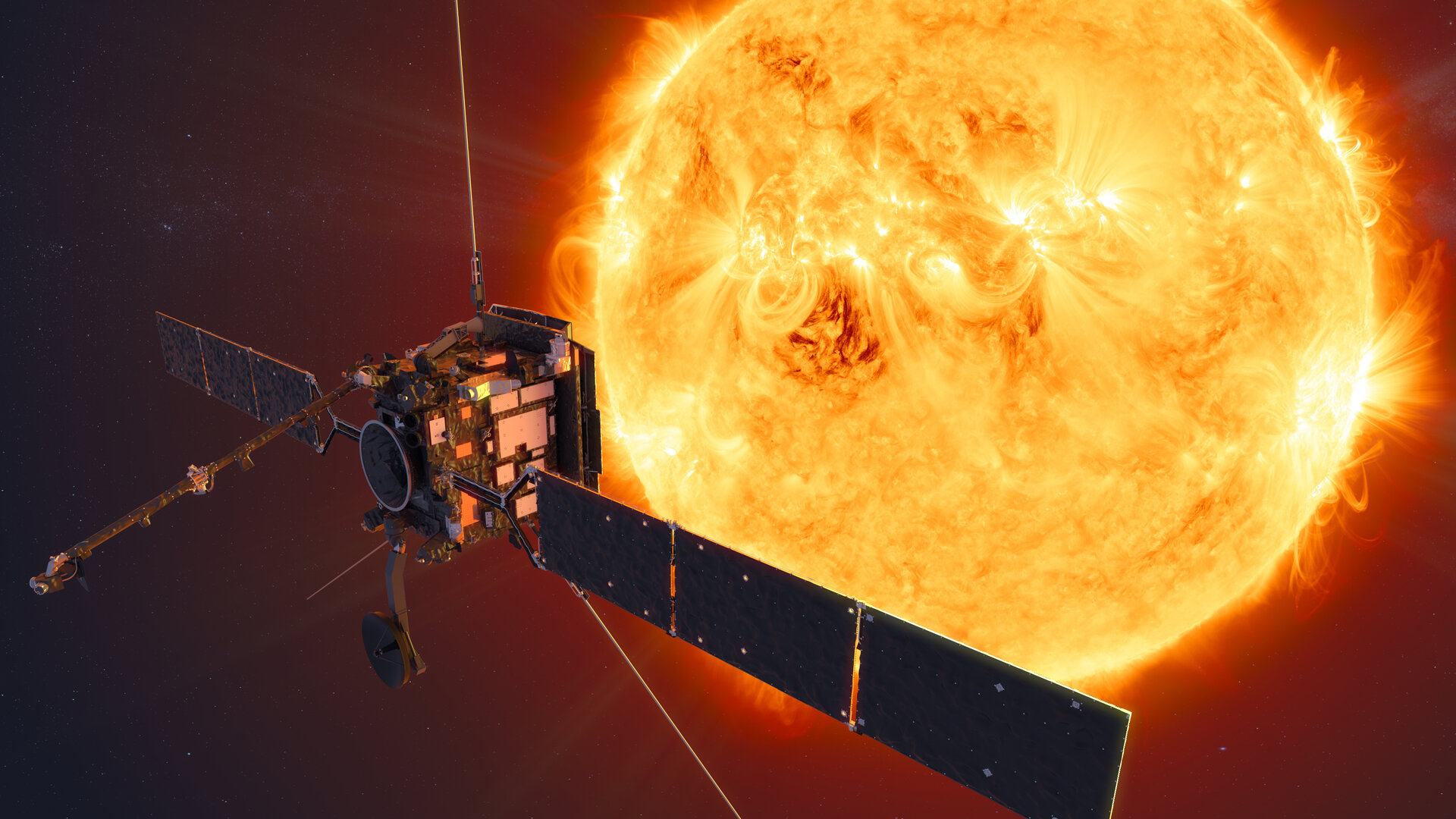
Access the video
Thanks to computer models, theorists now think that a flow of plasma from the equatorial regions of the solar surface to the poles sweeps away the decaying magnetic fields from old sunspots and other active regions. Once at the poles, these magnetic fields are submerged into the Sun and rejuvenated by the motion of the plasma at the tachocline. From there, they rise back to the surface to create the sunspots and active regions of the subsequent solar cycle.
At present, however, there is a lack of data on the distribution of the magnetic field at the poles because no spacecraft has yet seen the solar poles from close-up. Solar Orbiter will change this. By the end of 2025 the spacecraft’s orbit will be inclined enough that it will be able to target the solar poles with its telescopes and make these measurements for the very first time.
“The Sun’s poles are terra incognita,” says Sami. “It’s like the Earth 150 years ago, nobody had been on the poles, so there will be a lot of new things to learn.”
From helping to understand the generation of the Sun’s magnetic field, Solar Orbiter will also make unprecedented investigations of what happens once that magnetic field reaches out from the Sun and into space to create the heliosphere.
What heats up the corona?
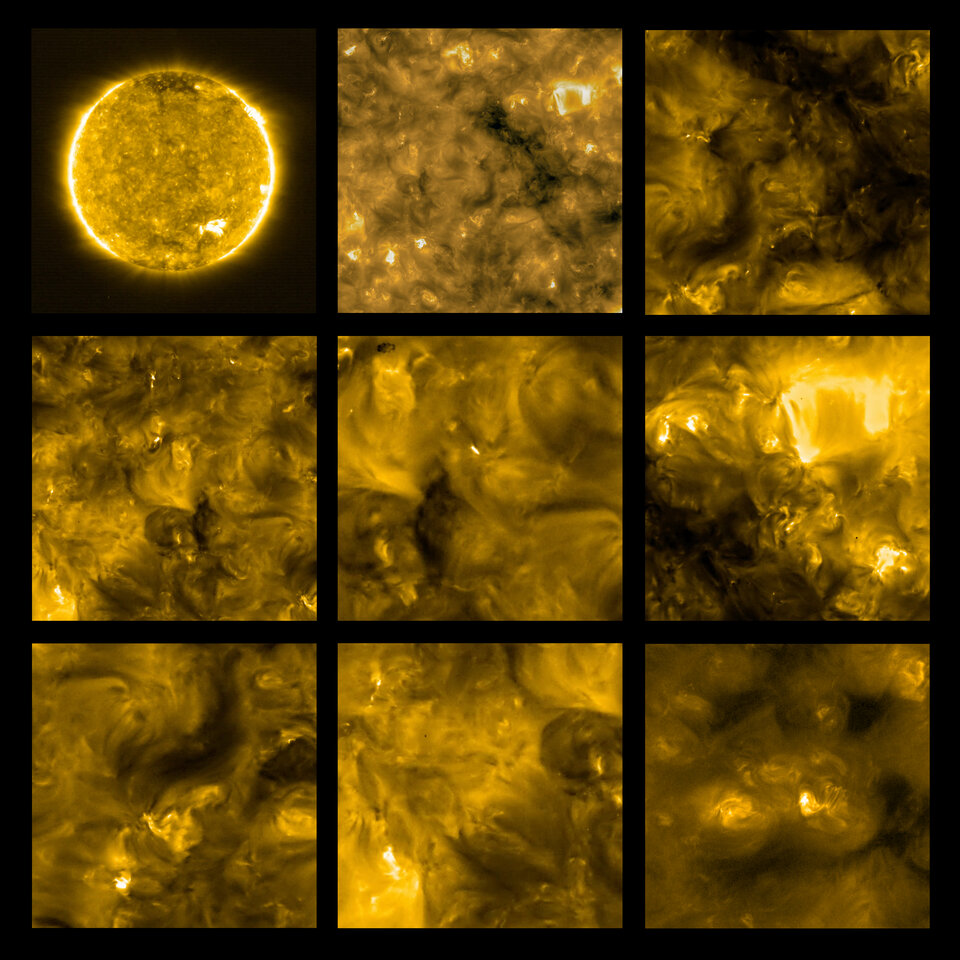
The Sun’s magnetic field is carried into space to create the heliosphere by a constant stream of plasma called the solar wind. This originates in the Sun’s outer atmosphere, the corona. And it is here that one of the great solar secrets is waiting to be uncovered: why is the corona so hot?
Somehow the tenuous outer atmosphere contains gas with a temperature of a million degrees, yet the solar surface is just 5500°C or so. This has been a fundamental mystery of the Sun since the 1940s.
“The fact that the Sun’s corona is so hot is quite counterintuitive,” says ESA’s Solar Orbiter Project Scientist Daniel Müller. “If you have a body that is very hot at the centre and relatively cool on the surface, it should be even cooler the further you go away. But the peculiar thing about the corona of the Sun – and other stars as well – is that it really starts to heat up the more you move away.”
Over the course of decades, there have been many plausible hypotheses advanced to solve this problem. Solar Orbiter will help to understand which of these ideas are actually at work. Many of them rely on releasing energy from the Sun’s magnetic field.
“When we look how much energy is in the magnetic field, and how much energy is in the particles [of the corona] we see there's about 100 times more energy in the magnetic field. If we release a little bit of magnetic energy, that means we can heat a lot of the particles, so that's what we try to understand,” says Säm Krucker, of FHNW University of Applied Sciences and Arts Northwestern Switzerland, and Principal Investigator of Solar Orbiter’s X-Ray Spectrometer/Telescope (STIX) instrument.
STIX looks at the solar flares, these are the biggest releases of magnetic energy that can take place. They can cause huge eruptions known as coronal mass ejections that eject billions of tonnes of particles into interplanetary space.
Other instruments look for smaller releases of energy that, while much smaller than the giant flares, may prove to be more important in heating the corona. This is because they take place more or less continuously whereas the large flares are generally few and far between.
Indeed, the first images taken by the Extreme Ultraviolet Imager (EUI) instrument, when Solar Orbiter was half way between the Sun and Earth, revealed that miniature solar flares, dubbed ‘campfires’ by the scientists, are an omnipresent occurrence near the surface of our closest star.
“The idea that the coronal heating might be caused by a vast number of tiny flares was put forward already in the 1980s by the famous US physicist Eugene Parker,” says Daniel. “It’s obviously too early to make any conclusions but it seems that Solar Orbiter may have just found a hint that Parker was right.”
What accelerates the solar wind?
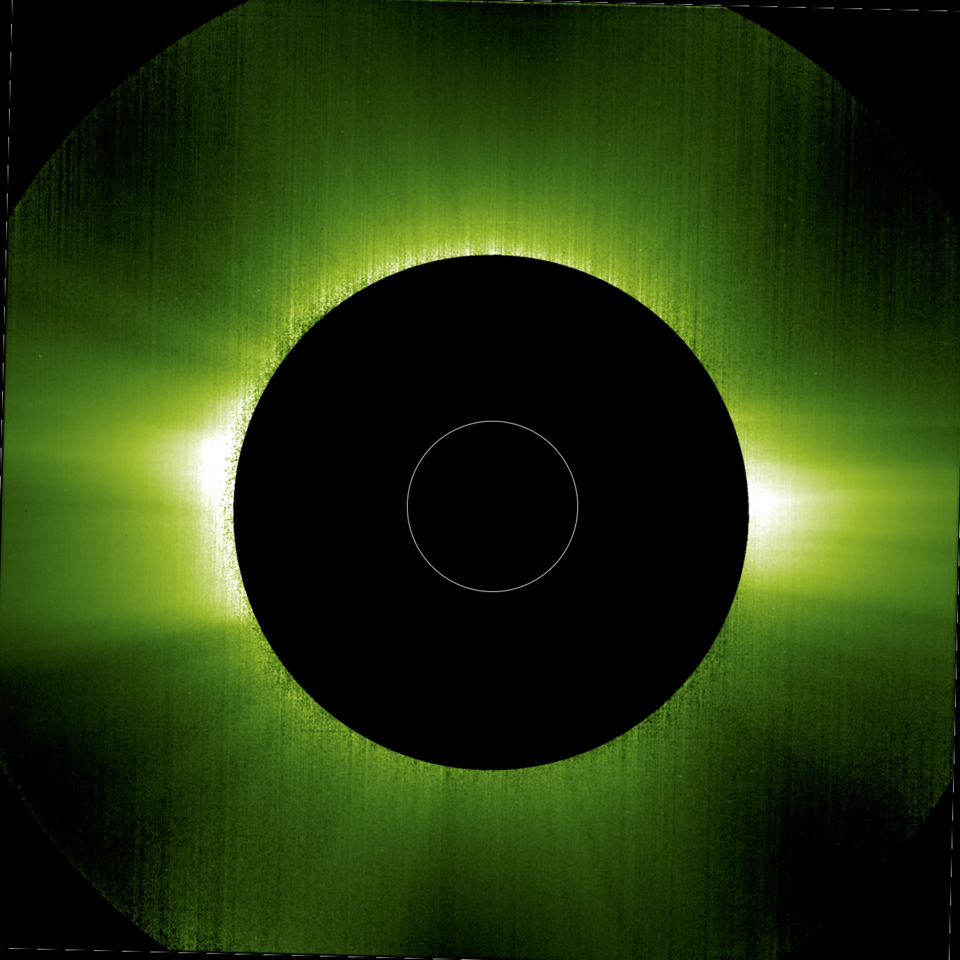
A related mystery to the heating of the corona is the acceleration of the ‘solar wind’, a constant stream of electrically charged particles that the Sun emits in all directions of space. The particles reach speeds of between 300 to 800 kilometres per second, but how they are accelerated to such speeds remains unknown.
“This is the big question for me,” says Tim Horbury of Imperial College London, and the Principal Investigator of Solar Orbiter’s Magnetometer instrument (MAG).
On the face of it, it seems simple. The sun's corona is really hot and so will naturally expand into space. But that isn’t enough to make the solar wind that we see. "It isn't enough to make it fast enough,” says Tim. So there must be some other acceleration mechanism at work.
To distinguish between the competing theories of how the solar wind is accelerated, Solar Orbiter will make very detailed measurements of its composition. Being so much closer to the Sun than the Earth, this data will provide information on the wind’s original composition at its origin point before any changes take place on it journey outwards through the Solar System.
“We are coming to realise that there are loads of variations in the speed of the wind, and huge variations in its magnetic field structure also,” says Tim. Any successful theory must therefore explain the enormous levels of complexity as well. It is a tall order, but one that Tim thinks Solar Orbiter can fulfil. “To my mind, the acceleration of the solar wind is the big question to answer. And I think we will do it, I really do,” he says.
The only star we will ever get close to
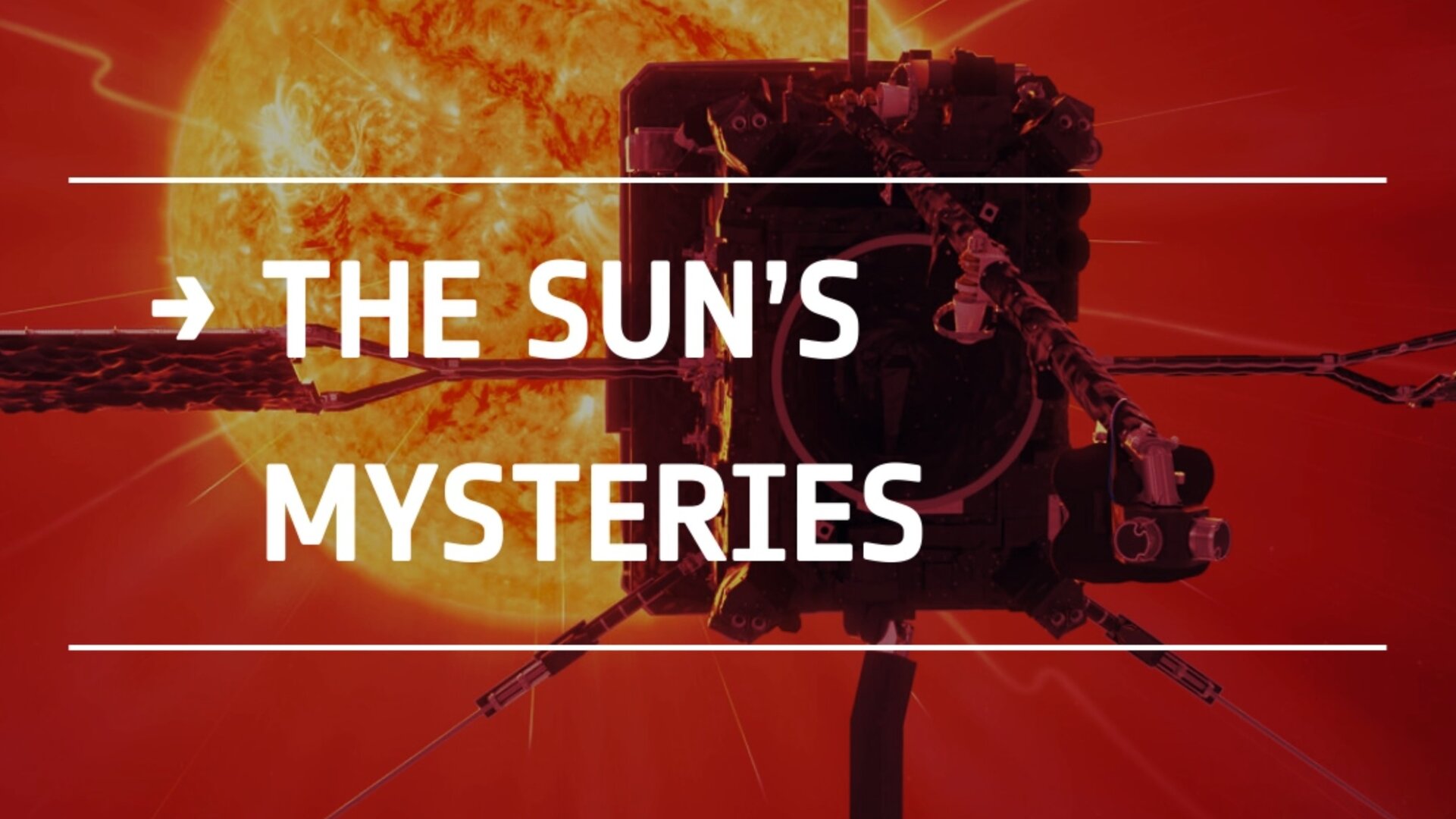
Access the video
As important as understanding our Sun is, the science of Solar Orbiter can be extended even further, and can be applied to our understanding of the wider cosmos. Magnetic interactions and particle accelerations can be seen taking place all over the Universe and at many different scales. Learning exactly how this happens by studying the magnetic field around the Sun will allow astronomers to apply that knowledge to many other aspects of the universe, such as star formation and the behaviour of matter falling into black holes.
For Yannis Zouganelis, ESA Solar Orbiter Deputy Project Scientist, this aspect of the mission holds great appeal.
“The reason I did solar physics is because the Sun - despite being a very ordinary star - is the only one we can really explore from close-up. And with Solar Orbiter, we can really go close and measure in-situ the properties of its extended atmosphere. For me, this has always been a way of doing space plasma physics that can be applied across the whole universe to many different phenomena. It is unique that you can plug in a physics equation for the Sun that can also be applied in exploding stars like supernovae and other very strange environments with plasma outflows,” he says, “And getting as close as we can to the Sun is really the only way that we can understand how it all works.”
Although the mission may be called Solar Orbiter, the science it will investigate impacts all branches of astronomy, and will fundamentally advance our understanding of key processes across the Universe. There are not many missions that can make that claim.