The wizards of orbits
Do you want to rendezvous with a comet beyond the Asteroid Belt? Or inspect the Earth at mid-morning every day? Then you'd better find out where to aim the rocket.
Once a spacecraft leaves terra firma, it enters a realm of perpetual motion where gravity steers it along the invisible tramlines in the sky called orbits. For figuring out the orbits that will take your spacecraft exactly where you want it to go, experts at ESA's European Space Operations Centre at Darmstadt in Germany know all the wizardry.
"Scientists and engineers come to us with their big new ideas and we fly them in our computers," says Walter Flury of ESOC's Mission Analysis Section. "We can then say not just what orbits are needed, but what launch windows and manoeuvres will achieve them. Our analyses also make plain the top weight of the spacecraft for a specified launcher, the onboard fuel requirements, and the ground stations needed for links. So all mission planning starts here at ESOC, with the orbits."
Circling around the Earth
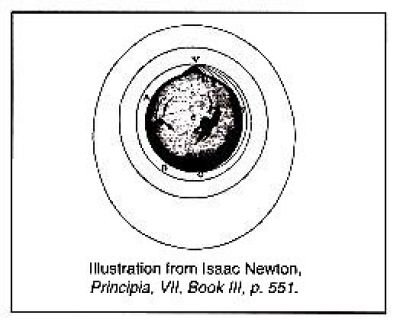
More than 300 years ago Isaac Newton pointed out that a powerful cannon on a hilltop could shoot a ball right around the world and back to its starting point - if only there were no air to resist it. At every moment, the ball falls towards the ground but it gets no closer because the Earth is round. The speed of the ball to accomplish this feat is 7.9 kilometres per second (more than 28,000 km/h) and 84.4 minutes elapse before an unwary gunner is hit on the back of his head.
In reality, air friction would make Newton's cannon ball glow like a meteor and drop to the ground. To survive, a man-made satellite must be lifted above the atmosphere. Big launching rockets are needed to fight the Earth's gravity, and the further out you want to go, the more work the rockets must do. Yet, once out there, a satellite need not travel so fast to maintain its distance from the Earth.
The International Space Station, for example, flies at 7.7 km/s in one of the lowest practicable orbits, at an average altitude of 390 kilometres. It circles the Earth every 92 minutes. Very thin air gradually makes it lose energy, and one of the tasks of the Automated Transfer Vehicles to be provided by ESA will be to nudge the Space Station from time to time, to keep it flying.
By going further out, and circling eastwards around the Equator, a satellite can match the Earth's rotation, in a 24-hour orbit. Then it seems to hover over the same point on the surface. This geostationary orbit, at an altitude of 35 786 kilometres and with a velocity of 3.075 km/s, is favoured for telecommunications satellites and for some weather satellites. There are already about 700 geostationary satellites in place. Of these 400 are no longer operational, and they contribute to a worrying increase in space junk and debris in the most popular orbits.
Putting a satellite into a geostationary orbit is nowadays a routine at Europe's tropical spaceport at Kourou in French Guiana, which is well located for the task. The Earth's rotation gives an extra boost to satellites launched eastwards, and the Atlantic Ocean provides a safety net in that direction from Kourou. A three-step sequence (1) injects the satellite into a low orbit, (2) boosts it into an elongated geostationary transfer orbit that carries it out to the right distance, and (3) boosts it again to the speed needed to remain at that geostationary height.
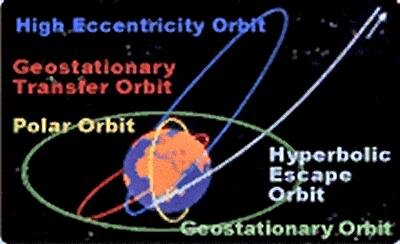
To visit everywhere in the world, select a polar orbit. For example, ESA's latest environmental satellite Envisat (2001) will fly at an altitude of 800 kilometres, and circle the Earth in 1 hour 41 minutes. It will not go exactly over the geographic poles. Instead the orbit will slant a little, so that it reaches to latitude 81.45 degrees north and south. By an effect of the Earth's shape, Envisat's angled orbit will then slowly swivel in space, at almost 1 degree a day, to match the planet's progress in its annual orbit around the Sun. As a result, on the sunlit side of the Earth, Envisat will pass over the regions beneath its track at about 10 a.m. local time every day and in all seasons. In contrast ESA's Cluster mission (2000) shows what happens if a polar orbit does not swivel as Envisat's does. The four Cluster satellites are on a 57-hour elongated 'high eccentricity' orbit that passes exactly over the poles and therefore stays pointing the same way, like a gyroscope. The farthest point of the orbit, 120 000 kilometres out, is within the tail of the Earth's magnetic shield in July but in January it is within the solar wind on the sunward side. So as the months pass, Cluster can examine the effects of the solar wind in different regions of the Earth's space environment.
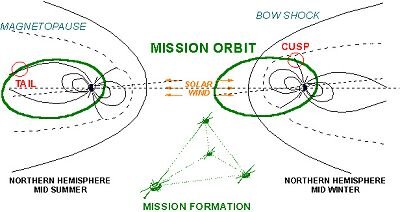
The four Cluster spacecraft, Salsa, Samba, Rumba and Tango, are all on slightly different orbits, and the distances between the spacecraft can vary from a few hundred to several thousand kilometres. Crucial for Cluster's unique 3-D view of events in the Earth's space environment is that the satellites should be in a triangular pyramid, or tetrahedral formation, in a region selected for investigation. This involves tricky speed adjustments, carefully computed to minimize the use of thruster fuel.
"When the idea of Cluster was put to us in 1984, the task of flying four spacecraft in a strict formation was the thing we looked at," Dr Flury recalls. "If that were not manageable, the project would not be feasible. No one had ever tried it before, but we satisfied ourselves that it could be done - as indeed the spacecraft operations team now demonstrates every day."
Into deep space
In Europe's first venture beyond the Earth's vicinity, an early Ariane launcher dispatched the Giotto spacecraft (1985) on a so-called hyperbolic orbit, which released it from the Earth's gravity and left it under the control of the Sun. Giotto thus became a diminutive planet. ESOC calculated its orbit in deep space so that it would intercept Halley's Comet in March 1986. When the spacecraft had survived a dramatic and damaging encounter, ESOC's mission analysts, flight dynamicists and spacecraft controllers were able to change its orbit to intercept a second comet.
Giotto's orbital change was achieved by 'gravity assist' when the original orbit brought the spacecraft back to the Earth's vicinity in 1990. Thanks to precise steering through the eye of a needle 22 731 kilometres above the Southern Ocean, Giotto changed course by 64 degrees and gained 3.1 km/s in speed, by the gravitational tug of the moving Earth. The new orbit achieved a perfect interception of Comet Grigg-Skjellerup in 1992. ESOC thus passed its first orbital tests in deep space with flying colours.
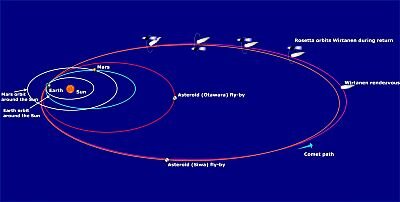
Swinging by the planets will enable ESA's Rosetta (2003) to alter its orbit not merely to fly past a comet but to match the comet's orbit around the Sun. Then Rosetta will be able to go into orbit around its target, Comet Wirtanen. Three planetary encounters, one with Mars and two with the Earth, will put Rosetta on course to rendevous with the comet far out in space. During its journey it will fly close to two asteroids. All of this is foreseen in ESOC's computers.
"No one can predict exactly how Rosetta should go into orbit around Comet Wirtanen," Dr Flury points out. "Only when the spacecraft gets there shall we determine more precisely the comet's mass and size. But the mass will be small, and the relative speed of Rosetta and the comet will be of the order of one kilometre per hour. It will be a delicate task for the operations team to fly the spacecraft to within a few kilometres of the comet, without crashing."
Hitherto spacecraft have followed ballistic paths, like Newton's cannon ball, except for occasional orbital changes using onboard rockets, or more delicate course corrections with small thrusters. Now, the computations at ESOC have to deal with solar-electric propulsion, which provides a small but continuous thrust throughout a long flight - first to the Moon with SMART-1 (2002) and then to the planet Mercury with BepiColombo (2009).
Another change concerns the 17th-Century mathematical laws of Johannes Kepler and Isaac Newton, which have faithfully served all spaceflight analysts since Sputnik-1. As Albert Einstein revealed in 1915, these laws are not quite correct. Any discrepancies have been far too small to affect spacecraft navigation till now. But the Einsteinian effects will become noticeable when ESA's Solar Orbiter (2009) approaches the Sun far closer than any previous spacecraft. To plan Solar Orbiter, ESOC's computer programs have been learning relativity.