Why we need Solar Orbiter
In the legend of Icarus, the boy’s father Daedalus builds them both wings made of wax and feathers so they can escape imprisonment on Crete. They fly across the sea, being careful not to get too close to the waves or the Sun. Yet, intoxicated by the god-like feeling of flying, Icarus flies ever higher until his wings melt and he plunges to his death. It is a fate that all who worked on the design of Solar Orbiter were determined to avoid. Indeed, the spacecraft’s outer heatshield is made to withstand temperatures of up to 500°C. While it is undoubtedly a great achievement to operate so close to the Sun, the question remains, why do something so risky with a €1.5 billion euro spacecraft when you can take images of the Sun from Earth? The answer, of course, is science.
The shortcomings of studying the Sun from Earth’s orbit
Until Solar Orbiter, most solar spacecraft have remained in the vicinity of Earth. For example, the Solar and Heliospheric Observatory (SOHO), the joint ESA/NASA mission launched in 1995, is in orbit around the Sun-Earth L1 point. This is a point in space, around 1.5 million kilometres closer to the Sun than Earth, in which gravitational and rotational forces balance so that the spacecraft can orbit the Sun at the same rate as Earth. This gives the spacecraft an uninterrupted view of the Sun. Others do not even go that far. In 2010, NASA launched the Solar Dynamics Observatory into an orbit around Earth.
While these missions have provided us with incredible insights and allowed scientists to develop excellent descriptions of the Sun’s behaviour, they have left us with fundamental questions to answer. Two of the key mysteries that Solar Orbiter is designed to answer is how the Sun heats its outer atmosphere, the corona, to a temperature of a million degrees; and how it accelerates the electrically charged particles that make up the solar wind. The designers of Solar Orbiter recognised that these mysteries can be addressed by getting closer to the Sun and immersing the spacecraft in the solar environment.
The desire to get closer to the Sun has been studied at ESA since the late 1990s. “It was a very ambitious mission concept,” says Daniel Müller, ESA Project Scientist for Solar Orbiter. And that meant it took a long time for technology to develop and be in place in order to make the mission possible.
Close but with no ‘eyes’

Access the video
Yet Solar Orbiter is not the first spacecraft to fly so close to the Sun. In 1976, the Helios-B spacecraft flew within a distance of 43 million km. Crucially, however, it did not carry any telescopes or cameras that looked directly at the solar surface. Instead it only sampled the particles and electromagnetic processes that could be found at that close distance to the Sun.
Neither is Solar Orbiter going as close to the Sun as NASA’s Parker Solar Probe. This spacecraft is currently shooting past the Sun at ever decreasing distances. Eventually, it is expected to pass by at a searing 7 million kilometres - six times closer than Solar Orbiter. However, the proximity means that Parker does not carry any cameras and telescopes to look directly at the Sun either because they simply would not work in the fearsome light and heat that the spacecraft will encounter.
Only Solar Orbiter, which is designed to operate at around 42 million kilometres from the Sun carries both particle detectors and telescopes. This unique combination will allow it to achieve its ambitious science goals.
Where the interesting physics starts
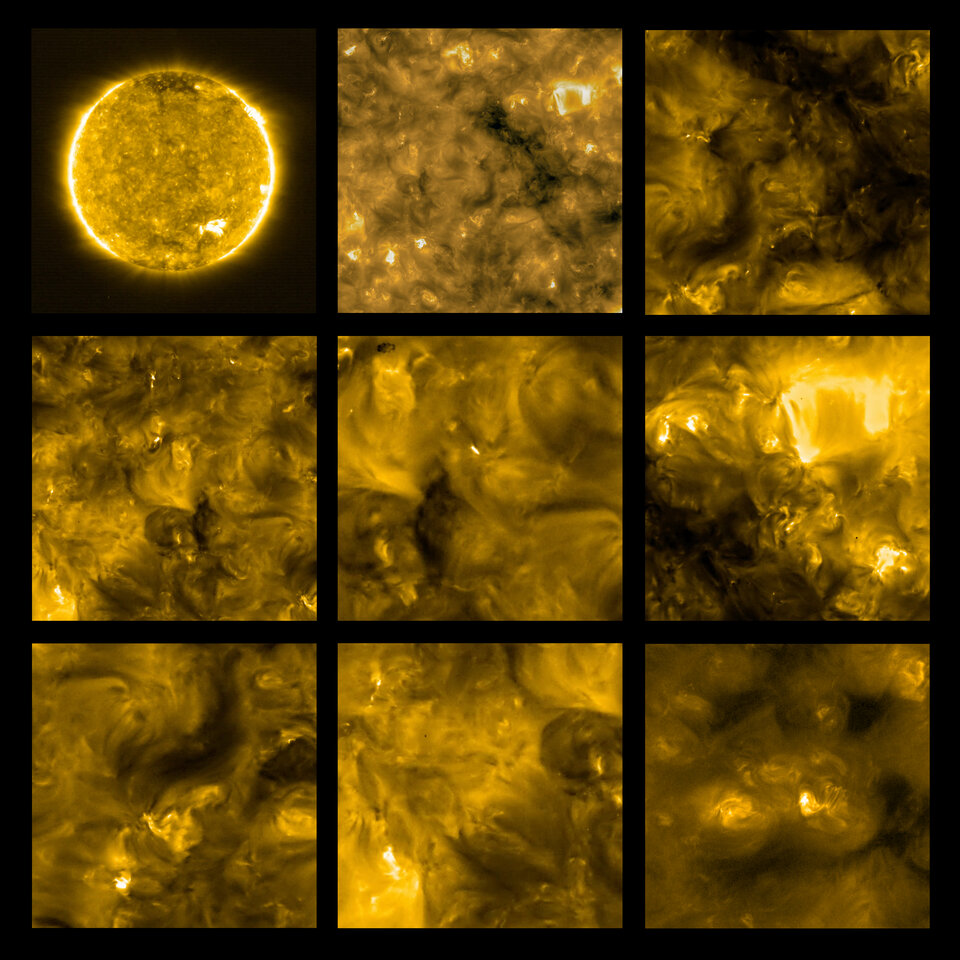
Getting closer to the Sun means that you can see it in more detail, and the closer Solar Orbiter gets, the more interesting the images and spectra from its remote sensing instruments are likely to be. During the commissioning of the spacecraft, the Extreme Ultraviolet Imager (EUI) instrument took pictures that revealed the surface was covered in what looked like miniature solar flares. And this is hopefully just a taste of things to come, says David Berghmans of the Royal Observatory of Belgium and the Principal Investigator of EUI.
“We hope to see ‘new’ features at smaller scales,” he says. He goes on to explain that the atmosphere of the Sun is ‘scale invariant’. This means that what happens on large scales, is mirrored by what happens in smaller scales. This is why EUI’s first discoveries were the miniature solar flares that the scientists dubbed ‘campfires’. At some level however, this self-similarity must stop.
“It cannot go down to the scale of an atom,” says David, “And the point at where it stops is where the interesting physics happens, such as coronal heating and the acceleration of the solar wind.” And understanding these two processes lies at the heart of Solar Orbiter’s objectives.
The detail and the big picture
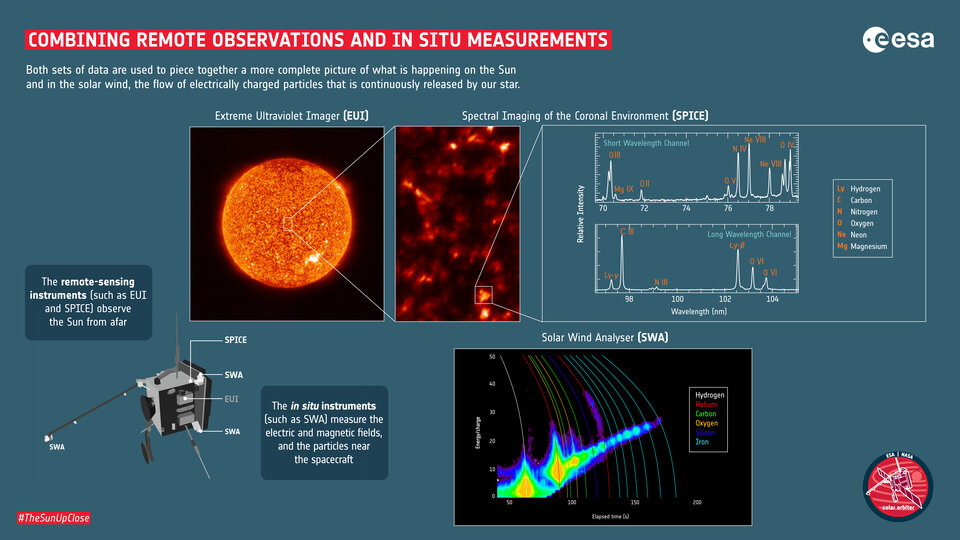
The interesting paradox for the mission is that although it is going as close as it can to the Sun in its quest for answers, it must also monitor the large scale so that it sees the way those surface phenomena affect the constant outpouring of the solar wind. It does this with the Metis and SoloHI instruments.
The coronagraph instrument (Metis) will take simultaneous images of the corona in visible and ultraviolet wavelengths. This will show the structure and dynamics of the solar atmosphere in unprecedented detail, stretching out from 1.7 to 4.1 solar radii.
The Heliospheric Imager (SoloHI) looks at even wider scales and takes images of the solar wind by capturing the light scattered by the electrons in the wind. Together these instruments allow the identification of transient disturbances in the solar wind, which can cause ‘space weather’. They trigger the aurora we see in the polar skies, and can have serious consequences for the technology we now rely on at Earth. The greatest space weather events are triggered by coronal mass ejections (CME). In these gigantic events, a billion tonnes of coronal gas can be ejected outwards and Solar Orbiter will be looking for these and other, smaller disturbances.
Putting everything together, the EUI detectors shows the coronal structures close to the solar surface and extending out into the Metis field of view, which then links to what is seen by SoloHI. Another instrument on Solar Orbiter, the Polarimetric and Helioseismic Imager (PHI) will measure the magnetic field structures near the surface of the Sun. This will allow scientists to look for the direct links between the behaviour of the Sun and the 'space weather’ that occurs throughout the Solar System.
“The combination of Metis, EUI, and PHI will be very powerful to point to the dynamics of CMEs that will be tracked by SoloHI,” says Russell A. Howard, of US Naval Research Laboratory, Washington, DC. and the Principal Investigator of SoloHI.
Observing the untangled magnetic field

In addition to the remote sensing instruments, Solar Orbiter also carries in-situ instruments that can detect particles and the magnetic field surrounding the spacecraft. When it comes to investigating the Sun’s magnetic field by collecting the particles that are being guided by it, only by going close can we ‘see’ the details of what’s really going on.
“At the distance of Earth, the Sun’s magnetic field is not made up of smooth lines like we are used to seeing around a magnet in the textbooks of secondary schools,” says Javier Rodríguez-Pacheco, of University of Alcalá, Spain, and Principal Investigator for the Energetic Particle Detector (EPD) instrument. Instead, by the time the Sun’s magnetic field has reached Earth, it can have become tangled and confused. This means that the Sun’s energetic particles, which are guided through space by the magnetic field do not necessarily point back to where they originated on the Sun.
“It means the picture that we have of these particles at Earth’s orbit is blurred,” says Javier. And this makes it impossible to understand the details of the energetic activity that ejects the particles because we can no longer be sure where they have come from on the solar surface.
Although the Sun’s light and heat are the most obvious connection we have to the Sun, the magnetic field and the particles it guides are increasingly important. Transient phenomena in these particles and fields are called space weather.
By going much closer to the Sun, Solar Orbiter will be flying through the magnetic field before it has been able to become so tangled. So using its telescopes and remote-sensing equipment, Solar Orbiter will be able to pinpoint the locations on the solar surface that are releasing the particles that are then flowing past the spacecraft and being detected by EPD and the other in-situ instruments.
Making the connections
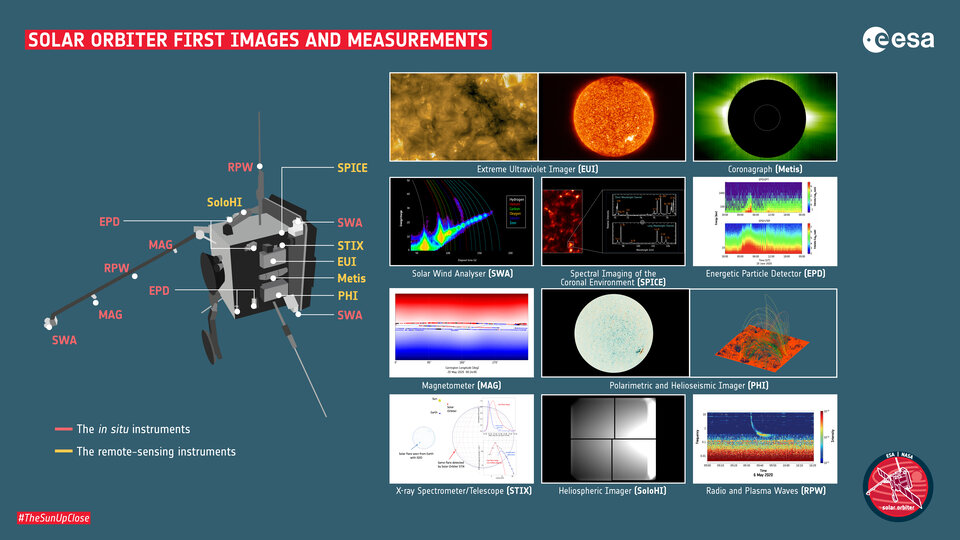
Of all of its investigations, this the key scientific goal of Solar Orbiter: to link what’s happening in space to what’s happening on the surface of the Sun. “This will be the unique thing that Solar Orbiter brings to the science; to say, ‘okay, we've seen what's going on on the Sun and now we’re seeing the outflow that's associated with that,” says Christopher Owen, of University College London’s Mullard Space Science Laboratory, and Principal Investigator of the Solar Wind Analyser (SWA) instrument.
An important part of this investigation will be performed by SWA’s heavy ion detector. Heavy ions are atoms like oxygen or carbon or iron that have had electrons removed, rendering them electrically charged. The exact charge carried by these ions is related to the number of electrons removed from the atoms, which is determined by the temperature and density of their source region in the corona. What makes them scientifically important is that their electrical charges do not change as they flow outwards in the solar wind. “They are a direct link back to what's going on on the Sun. They will provide a very important fingerprint for us to compare to what the remote sensing instruments are seeing, and so identify the specific source regions and mechanisms,” says Christopher.
The Spectral Imaging of the Coronal Environment (SPICE) instrument will make one of the key comparisons. SPICE measures the chemical composition at the surface of the Sun, which can then be compared to the composition of the solar wind measured by SWA. If the campfires really are the regions that provide the energy to heat the corona and accelerate the solar wind, then SPICE will show that the composition of plasma near a campfire is the same as that of plasma which subsequently flows past the spacecraft and is detected by SWA and other instruments.
“And then we should be really in a position to measure the density of ejected material and the outflow velocity,” says Daniel. By doing this, Solar Orbiter’s scientists could identify the source regions, and therefore the acceleration mechanisms, of the various streams in the solar wind. “If we can really say, ‘the spacecraft is now passing through a big stream of particles that came out of this particular active region,’ that would be fantastic. That is something that I personally would really love to see,” Daniel adds.
“For the first time, we will be able to link these two worlds and understand the mechanisms that are accelerating the particles, and how they propagate in the Interplanetary Magnetic field,” says Javier, “We should be able to use this to calculate the particle fluxes and directions at other points of the solar system. I think that this will be remarkable.”
In short, Solar Orbiter represents end-to-end science in a way that’s never been done for the Sun before. It is a big, bold mission with a clear ambition – and a lot of fascinating work ahead of it