Here there be radiation dragons
‘Here there be dragons’ – medieval mapmakers would daub such warnings on dangerous or unknown recesses of mariners’ charts. Fast forward to the 21st century and the space surrounding our planet holds ‘dragons’ of its own: fierce radiation belts that surround Earth, Jupiter and other planets, and storms of particles originating from the Sun that travel far into space. A dedicated class of engineers work to safeguard space missions from such dangers.

ESA radiation effects engineer Christian Poivey has been working in this field for more than three decades, last year receiving the annual Radiation Effects Award from the international Nuclear and Plasma Sciences Society.
“When I first got started, I knew very little about the subject,’ Christian recalls. “It was fascinating to find out about the radiation belts around Earth, the Van Allen belts, and how electrical devices could be impacted. I trained as an electrical design engineer, receiving my PhD in 1988, but when I was recruited to Matra – now part of Airbus – my job included assessing radiation effects as well as designing integrated circuits for space.”
He went on to spend eight years working at NASA before returning to Europe to join ESA, giving him a good perspective on the international radiation community – his award highlighting his skill at working across borders. Radiation effects are indeed a serious concern for all space agencies.

Our radioactive cosmos
Space is inherently radioactive. High energy particles are fired our way from across the wider Universe – known as Galactic Cosmic Rays. Protons are also emitted from the Sun during occasional but violent flares or Coronal Mass Ejections.
Earth’s magnetic field protects the surface from most of these charged particles, but some get trapped within two doughnut-shaped rings around the planet, known as the Van Allen Belts after their discoverer, space scientist James Van Allen, who oversaw the first US satellite, Explorer 1, in 1958.
The inner Van Allen belt starts at about 600 km from Earth’s surface, with the outer belt about 65 times further away. But satellites in much lower altitude orbits are also vulnerable, because of a kink in Earth’s magnetic field that brings part of the belt much closer – known as the ‘South Atlantic Anomaly’.

Satellites in orbit are much more likely to experience faults in the vicinity of this region. For instance, in 2016 Japan’s Hitomi X-ray telescope mission was lost entirely due to multiple errors in its attitude control system, believed to have been triggered by radiation effects.
“ESA has never lost an entire mission due to radiation, although individual instruments have failed in the past,” explains Christian. “For instance the PRARE ranging sensor on the ERS-1 Earth-observing mission was lost just five days into flight, probably to radiation-driven onboard memory damage. It is the job of radiation effects engineers to try and make sure this kind of loss does not happen!”
Dealing with – not avoiding – radiation effects
When a charged particle impacts a component, the immediate effect is something called a ‘Single Event Upset’, a brief electrical discharge that might lead to random glitches, memory flips or possibly ‘latch-ups’ – localised short circuits. The accumulation of particles received by a component is the ‘Total Ionising Dose’, which causes a gradual degradation of functionality as radiation exposure builds up internal defects within a part. Incoming particles also displace atoms in the crystal lattice of semiconductors – called ‘Displacement Damage’. Both effects cause gradual degradation of the electrical performance of components.

“For these gradual effects, the best solution is to build extra margins into the design, guided by the planned mission life, to maintain desired functionality,” adds Christian. “For Single Event Upsets it is not possible to design fully radiation-hardened components to entirely avoid the problem. Beside being heavy, and ineffective when it comes to fast, heavy Galactic Cosmic Rays, shielding around components could also give rise to showers of secondary particles that might actually do more damage.
“Nowadays, in a change of paradigm we seek to deal with this problem instead of sidestepping it. One strategy involves redundancy, where data is stored in multiple locations across an integrated circuit, so it cannot be entirely corrupted by an individual bit flip. Redundancy also means that differing memories can cross-check with each other and vote on the most likely result. Or when an overcurrent is detected, likely due to radiation, then current can be shut down to avoid a latch-up or other permanent damage.”
Trial by particle accelerator
The good news is that, in general, modern components are proving less susceptible to radiation effects. The job includes the practical radiation testing of components using particle accelerators and other radiation facilities more typically used for cancer treatment and other types of nuclear medicine.

“In general testing is vital, especially for Single Events,” adds Christian. “Field Programmable Gate Arrays, for example, have become so complex that accurate predictions of radiation effects are not possible without testing. You can make all the plans you want, but when you start trying them out for real you’re always going to be surprised. What you end up with is a representative ‘error signature’, so you can accurately predict what kind of malfunctions will occur in space, and how often.
“There’s also increasing interest in the use of ‘commercial off the shelf’ parts compared to traditional high-reliability radiation tolerant parts to reduce the cost and increase the performance of missions. The issue here is that these have no radiation countermeasures designed into them, so their reliability is much lower. And there is limited documentation for traceability, to see precisely how the parts have been put together and from which materials. Practical radiation testing becomes even more important in such cases, because it turns out that different component batches can have very different radiation responses, so it can be very useful to pre-screen them accordingly.”

Challenging missions, from Hubble to Juice
The missions that Christian has supported during his time included the fourth and final servicing mission to the Hubble Space Telescope, whose repeatedly troublesome gyros are most likely due to radiation effects, and the mirror deployment of the James Webb Space Telescope in the relatively benign deep space environment of Lagrange Point 2, 1.5 million km from Earth.
This contrasted with the extreme challenge of ensuring ESA’s Juice mission was sufficiently radiation-hardened, since the gas giant is bounded by its own highly energetic equivalent of the Van Allen belts, making it the most radioactive environment in the Solar System.
“Getting around this involved placing all of the electronics in a lead-lined vault,” Christian explains. “There was also much more calculation involved than a standard mission, to estimate the total radiation dosage to be received by each and every component.”
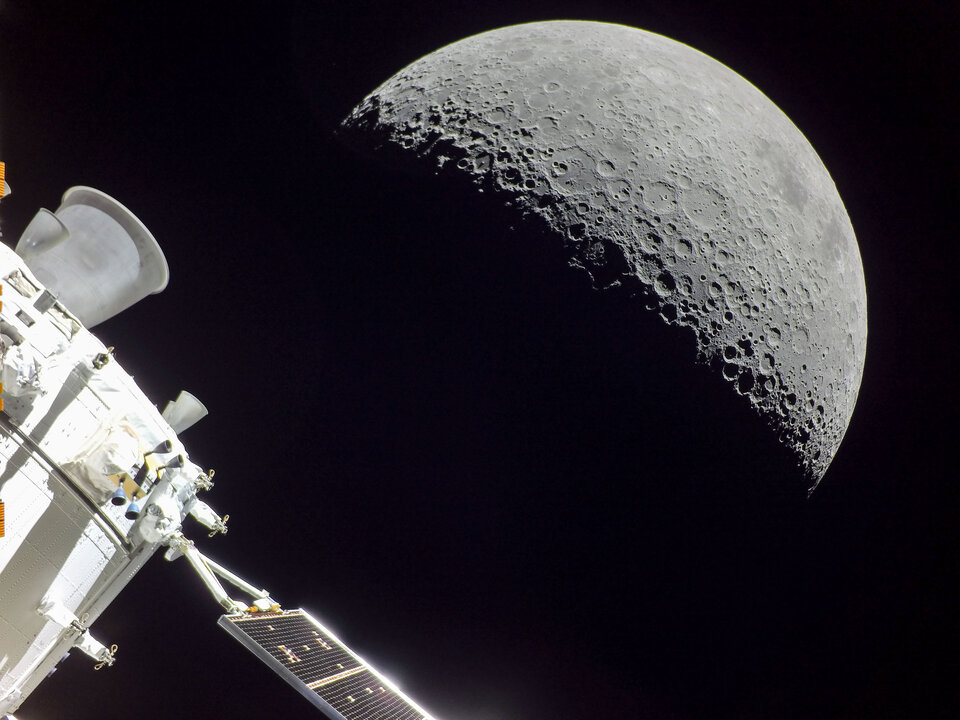
Christian has also worked on the NASA-ESA Orion lunar spacecraft and the Metosat Third Generation series of European weather satellites.
On the ground he supported ESA’s Microelectronics section during their development of radiation tolerant components such as highly customisable BRAVE (Big Re-programmable Array for Versatile Environments) Field Programmable Gate Arrays as well as the LEON-5, the latest multi-core generation of Europe’s general purpose integrated circuits for space.

Coming next
Future missions he has been engaged with include the GENESIS satellite which needs to orbit at 6000 km altitude to produce an improved reference frame of Earth – deep inside the inner Van Allen belt – and the LEO Cargo Return Service to take cargo to and from space stations in low-Earth orbit, as well as the Harmony Earth Explorer mission to detect small-scale motion on Earth’s surface.

Looking towards the future, radiation hardening is becoming increasingly relevant on Earth as well as space. A cloud chamber operating near Christian’s office at ESTEC in the Netherlands makes clear the amount of charged particles from space that make it down to the ground. Computer data centres concentrate together so many electronic components so densely that radiation from space has become a statistically significant source of errors.
“The next time your computer crashes, it might possibly be due to space radiation,” notes Christian. “That might not be such a big deal, but it could be when you scale up to safety-critical systems such as those aboard aircraft or autonomous vehicles that need to go on working reliably on a continuous basis – meaning that radiation-hardness is only going to become more important in both space and down on the ground.”